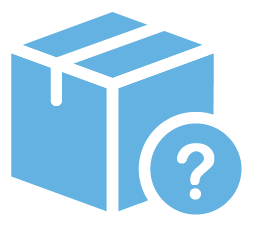
Need help choosing the right product?
Our tool will match the best product to your needs
Launch product selectorWelcome to the USA Website
We have detected that you may prefer the Global site. Please use the language dropdown above to change your selection if required.
When sensor signals are disturbed by an external power source you can expect measurement errors and process malfunctions. We will look at the mechanisms and principles behind electrical interference and point out some preventions and cures.
Interfering sources include: nearby power cables, motors, transformers, radio transmitters, variable speed drives and discharge lighting.
The offending sources deliver leakage currents through capacitance and imperfect insulation and spray magnetic and electric fields at your signal wiring. The resulting injected voltages or currents can overload and paralyze signal amplifiers.
Thermocouple and RTD signals, being at low dc millivolt levels are the most susceptible, so I will use these in examples that follow.
Leakage Resistance allows both alternating and direct currents to enter signal circuits.
Capacitance allows an alternating current to enter the signal circuits. The value of this current is: capacitance times rate of change of voltage.
(formula i = C dv/dt or I = VωC for sinusoidal ac interference of frequency f ) ω = 2πf
Your defenses are:
1. High insulation resistance: measure it and upgrade if it gives trouble.
2. Distance: keep the signal wires away from power wiring. Both are better in their own
conduits or ducts. These measures will minimize resistive and capacitive currents.
3. Shielding Keep signal wires inside a metal shield which is grounded at one point only. This diverts both resistive and capacitive currents harmlessly to ground.
Changing magnetic fields from power cables or equipment can link the signal wiring. This induces a voltage which is the product of the mutual inductance M between the interfering source and the signal wires and the rate of change of the interfering current i
(formula v = Mdi/dt or V = IωM for sinusoidal currents of frequency f ). ω = 2πf
Conductive shielding is ineffective here and even magnetic shielding is of limited use. Separation of signal and power wiring is again the best measure.
Twisted pair signal wiring: This acts by successive twisted loops canceling each other’s pick-up of magnetically induced voltages (reduction of mutual inductance M).
When you have taken all the above measures you could still be left with some unwanted voltage, effectively in series with the signal. This is called Series Mode interference and is usually ac. Occasionally it could come in dc form. E.g. leakage currents in electrolytic tanks or a bare thermocouple clamped to a conductor carrying direct current. In the dc form, prevention by good isolation is the only remedy since you cannot filter out dc from a dc signal.
A low-pass filter will reduce ac interference. Such filters can be combinations of capacitors, resistors and inductors in the signal lines or they can be digital filters built into the controller’s electronics. Their job is to allow passage of the dc process signal and attenuate (reduce) the interfering ac. Such filters increase the response time of the controller or indicator to changes in signal level. Since temperatures don’t change all that fast, this is not usually a problem. If you are scanning multiple inputs into a time-shared instrument containing a filter you may have to put up with a slow scanning speed in order to wait out the settling time of the filter as it acquires each dc signal. Alternatively you could use a filter on each input.
Modern controllers and indicators use another technique to deal with the remaining ac superimposed on the dc signal. They digitize the average dc signal over a period that contains an equal number of canceling positive and negative half cycles of the ac interference. This means that the interfering frequency has to be known by the digitizing process. Usually 50 or 60Hz is anticipated.
We now come to series mode and common mode definitions and isolation techniques.
Common Mode voltage means that both terminals of the signal are elevated in voltage relative to ground. For example a thermocouple could be welded directly to the live (i.e. not neutral) end of an ac heater. The ac voltage at this point relative to ground is called the Common Mode voltage.
Instrument input circuits are usually designed to cope with sensors that are connected to sources that could be up to some 250V above ground. This elevation can be either an unavoidable process requirement or by accidental contact with a live electric heater.
In the presence of common mode there is always some resistive or capacitive leakage-current to ground from each signal wire and the leakages are not necessarily equal. These currents pass through the two thermocouple conductors – also unequal in resistance. The difference in IR volt-drops in these conductors can produce a series mode interference component which you can deal with as above.
Instrument manufacturers design to minimize the effect of interference and specify a Series Mode Rejection Ratio, defined as:
The value of the series mode interference voltage/the error in indication that it causes – both expressed in volts.
You will see rejection ratios typically of 103. In this example 1V of series mode interference would cause a 1mV measurement error.
Likewise the Common Mode Rejection Ratio is:
The value of the common mode voltage/ the error in indication that it causes – both expressed in volts.
For common mode you will see numbers in the order of 108. In this example 100V common mode causes 1μV (one microvolt) of error.
Instead of publishing these simple numerical ratios it has long been the custom in manufacturer’s specifications to convert them to decibels. 103 converts to 60dB and 108 converts to 160dB. To convert this weird unit back again so you can work with it, divide the dB number by 20 and use it as the exponent of 10.
One or two millivolts of interference is all it takes in a thermocouple circuit to spoil your work or equipment. On the up side, the low source resistance of thermocouples (a few tens of ohms) makes the signal more robust against interference. That is, until you start adding cable length and resistance to reach a distant control panel. At this stage you are also presenting a receiving antenna to interfering sources.
Sensor types that deliver larger voltage signals are correspondingly less vulnerable. However some can have source resistances of several kilohms, even megohms and for this reason present an easy target for interfering sources.
There are times when you have done all you can with wiring layouts, twisting and screening and you still want to protect vulnerable signals and long wire runs. There is a good case for inserting a signal converter close to or even on the sensor.
The output of a typical thermocouple converter is commonly a robust 4 – 20mA signal, both linearized and cold-junction compensated. You wire its clean, isolated output in cheap copper cable and the signal
arrives unchanged at the controller or indicator.
A few steps up from signal converters takes you to a digital control and data acquisition system. Here each sensor signal enters its own controller just as it would enter a signal converter, at or near to the source of measurement on the process. Instead of a dc output, digital information derived in the controller now goes on a common bus to the control station or human machine interface (HMI), fast and without degradation. These signals represent for example, process variable, one or more set points, alarm condition, control parameters, controller output. Local display and local heat output (e.g. built-in SCR ) can be optional.
In the present industrial environment you can expect severely non-linear and fast-changing electrical loads such as high current arcs, variable speed ac drives, switching power supplies and phase-angle SCRs.
These are major causes of distorted waveforms on your power line instead of the docile, smoothly changing sine waveforms we usually take for granted. When you power your instrumentation from such supplies you may encounter process upsets caused by corruption of the internal digital circuitry. Some instrument manufacturers are better than others and severely torture-test their products as part of the design process and ensure that they are virtually immune to bad power quality and electromagnetic interference. You may suspect your power quality or see on your oscilloscope a distorted and spiky waveform. If you do, consider using a voltage stabilizer or power conditioner of the kind that also cleans up the waveform (i.e. renders it sinusoidal). There is a wide range of offerings bearing these names. Some are effective some are useless so you will have to read and understand the specifications and ask questions.
Power quality problems lead some distributed control equipment manufacturers to use a stable and clean low-voltage dc supply to power the equipment.
Product Selector
Our tool will match the best product to your needs
Launch product selectorContact Us